
Identifying and measuring discharge events in high-voltage ceramic feedthroughs
The role of ceramic-to-metal seals
High-performance sensors built for harsh environments typically include some type of ceramic-to-metal seal. These hermetic seal assemblies come in a wide range of sizes and configurations. These seals play a particularly large role in gas-filled neutron and x-ray detectors, including ones for gamma scintillation.
Ceramic-to-metal seals and feedthroughs provide a hermetic boundary that allows power and signals to transmit to and from the gamma detectors used downhole during drilling operations. While these detectors have a good yield, they often can have difficult-to-trace seal issues that cause poor performance at high voltages and temperatures in these applications.
There are ways, however, to identify and remediate these issues, as we discovered during routine manufacturing testing at Reuter-Stokes.
Anatomy of a gamma-ray detector
Natural gamma-ray detectors are used to detect radiation around wellbore formations. They are part of the formation analysis detector suite of the drill string’s bottom assembly. These detectors consist of a photomultiplier tube, a thallium-doped, sodium-iodide crystal, a stainless-steel, outer-body tube and end cap, suspension springs and washers, and a four-pin, ceramic pass-through, hermetic-seal assembly. The entire device is built inside a glove box with dry nitrogen that has less than 1% relative humidity. It’s welded into a completely hermetic assembly that operates at temperatures of up to 150°C, at up to 20gRMS vibration.
Examining poor detector performance
During routine testing of gamma detectors in settings where temperatures swing from room temperature to 150°C, our testing team began to encounter failures, including noise and overall poor detector performance.
We determined excessive count rates from the detectors caused these issues when testing occurred in temperatures higher than 125°C. We couldn’t duplicate the issue at room temperature.
The team launched root-cause analysis by eliminating various components in the gamma-ray detector assembly. Between that and other efforts to “workaround” the problem, including computational modeling, we uncovered the offending component: the hermetic seal assembly that welds into one end of the stainless steel tube.
We learned that when we used proper wiring, we could bypass applying ground potential to the weld ring on the seal assembly’s exterior and eliminate the issue in mocked-up detectors that exhibited the failure mode. We could turn it on and off like a light switch. We knew we had the right component but needed to dig deeper into why these issues occurred with the hermetic seal.
Troubleshooting the discharge events
Considering the nature of the sensing electronics used in the gamma module, we determined the problem didn’t stem from direct-current leakage between the high-voltage bias on the PMT output and the grounded weld ring. Because the PMT signal is AC coupled through a capacitor to the detector’s charge-sensitive preamplifier circuit, a DC leakage current couldn’t pass the coupling capacitor.
Using electromagnetic modeling in COSMOL, engineering insight, and a little luck, we determined we were seeing partial discharges in the seal assembly. There had to be impulsive charge transfers somewhere in the seal system to be able to mimic the output of the PMT.
It appeared the air gap between the inner wire and the inside diameter of the ceramic body, adjacent to the braze joint at the seal assembly’s outer metal shell at the outside of the ceramic insulator, was where the highest likelihood of discharges could occur. We had no visual means to observe this, so we had to find a way to measure the charge transfer and quantify it with regard to detector electronics’ sensitivity to assure correlation.
As such, we decided to treat this seal assembly like a gas-filled detector, one filled with air at atmospheric pressure.
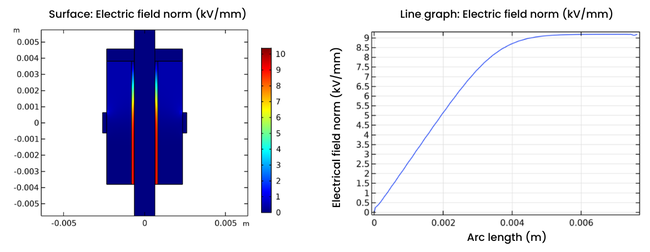
We designed a test system of components used in our manufacturing processes to treat it as such.
The measurement system primarily used off-the-shelf electronics, an oscilloscope, a multi-channel analyzer (MCA) and a laptop for data acquisition and analysis. Because the lower threshold for pulse discrimination in a detector is on the order of 1 pico-Coulomb, we also used a charge-sensitive amplifier.
In a normal proportional counter measurement system, the signal would be sent to a counter/timer with a specific threshold-level setting. Any discharge that created voltage from a shaping amplifier above a specific value would be counted as a valid pulse. In our application, we had to be able to quantify the individual charge transfers so that we could correlate them to the response of the product electronics in the production environment.
We calibrated test systems measurements using an air capacitor and waveform generator. These injected a known charge into the electronics to characterize a response, which we compared to the production electronics and verified was in agreement.
We measured the discrete pulses generated by discharges across the air gap between the conducting wire and the coaxial ceramic insulator at 1600V and 150°C ambient temperature. We discovered configurations where signal and high voltage were present on the same feedthrough, voltage breakdown of the gas filling the gap between the wire and insulator could produce charge pulses that are indistinguishable from the output of the photomultiplier tube.
The team placed samples in an environmental chamber set to 150°C and measured each sample pin individually. Each pin was energized with 1600VDC. After the samples reached temperature, each discrete pulse was amplified and shaped. The MCA, the key to the testing approach, then recorded the shaped pulse’s amplitude for about five to 10 minutes for each pin. We plotted pulse-height distribution as a histogram of counts versus pulse charge.
The process revealed “good” and “bad” pins with different pulse-height distributions. The count rate attributed to noise for the pins at amplified temperatures was <0.1 counts per second (cps). The bad pins would have generated many counts per second at elevated temperatures. The presence of excess noise only at high temperatures and high voltage yielded temperature-dependent noise count rates. This led to unacceptable count-rate stability for the detector system.
Final analysis: a breakdown
As we reviewed the histogram, we noticed that the pulse-height distributions generally had non-exponential shapes. Furthermore, the distributions seemed to exhibit some charge quantization with a peak at approximately 15 pico-Coulombs. This has a statistical significance of 4.8σ above a smooth background hypothesis. Some of the pins also exhibited a broad distribution that wouldn’t be characterized as peaks or quantization.
We have drawn the following conclusions from this testing:
1. The quantization is related to the details of each pin’s conductor-insulator geometry, as well as the insulator’s surface and bulk resistivity.
2. The conductor-insulator geometry varies significantly on a pin-to-pin basis and may impact the seal’s observed quality.
3. Factors affecting surface and bulks resistivity and a localized increase in the electric field are presumed to determine seal assembly performance in high-temperature and high-voltage.
4. The effect can be measured in a straightforward way using the appropriate instrumentation suite.
5. Hermetic ceramic seals can be screened for quality using this process.
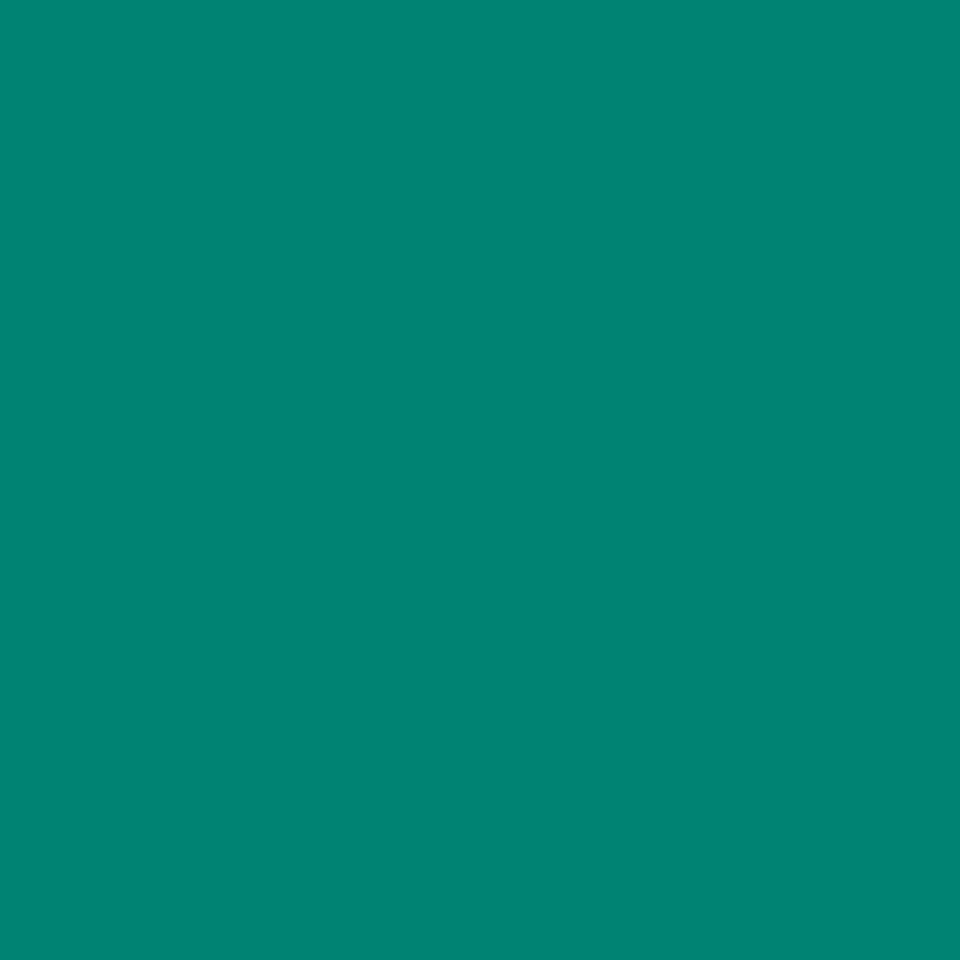
Reuter-Stokes was granted a patent on this methodology following our research and testing. Further, that patent was then used to develop a methodology for reducing discharge within the sealed environment of the detector, earning Reuter-Stokes another patent!